We study fundamental aspects of quantum mechanics, interactions and elementary particles along with quantum systems manageable in the lab in view of next coming applications.
The activities at FIM are focused on:
- Quantum Spintronics
Molecular Magnetism
Molecular Quantum Spintronics
Circuit Quantum ElectroDynamics
Quantum Dots based on semiconductor nanowires
Superconducting qubits and photon detectors - Quantum Electron Microscopy
Single protein detection
EMCD (electron magnetic dichroism) at atomic resolution
Plasmonics - Modeling and Simulations of Solid-state Devices for Quantum Technologies
Electron quantum optics: dynamics of electronic interferometers
Quantum walks on graphs for quantum information and computation
Coherent dynamics of interacting quantum complexes
Charge and spin excitations in quantum nanostructures for qubit deployment - Quantum Field Theory and String Theory
Gauge/string duality
Supersymmetric gauge theories
Worldline approach to QFT
Anomalies in QFT - Experimental Physics of Fundamental Interactions
search for new particles
antimatter production in high-energy proton-nucleus collisions
research and development of future detectors
Faculty
Marco Affronte, Marco Beleggia, Andrea Bizzeti, Paolo Bordone, Olindo Corradini, Stefano Frabboni, Guido Goldoni, Diego Trancanelli, Francesco Rossella, Enrico Bertuzzo, Marco Govoni
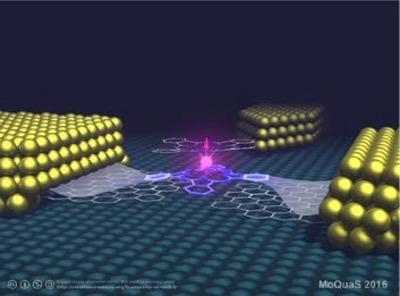
Molecular Quantum Spintronics is the art of combining magnetic moments with charge currents or photons in the quantum regime, that is controlling molecular levels, superposition and correlation of states.
Our group devises hybrid devices combining selected molecular spins with graphene conducting layers of superconducting circuits to exploit genuine quantum features of molecules.
The experimental facilities include cryo-magnetic systems with advanced nanoelectronic and microwave equipment.
- Molecular Magnetism
- Molecular Quantum Spintronics
- Circuit QuantoElectroDynamics
- Quantum Dots based in semiconductor nanowires
- Graphene-based devices
- Superconducting qubits and photon detectors
Image credits: MoQuaS and FIM, UNIMORE
People: Prof. M. Affronte; Dr. A. Ghirri (CNR); Dr. C. Bonizzoni, Dr. M. Govoni (CNR)
Research group website: www.lowtlab.unimore.it
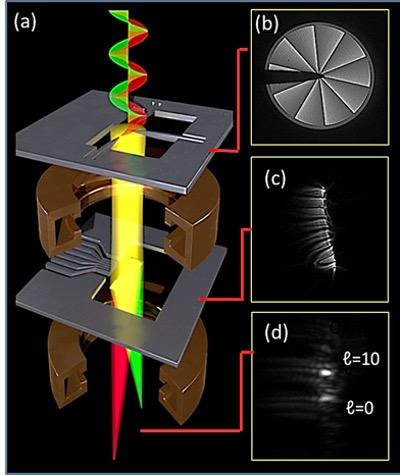
The measurement in quantum mechanics is always associated to the collapse of the wavefunction. The loss of information in the measuring process implies that measurements of physical quantities are obtained averaging over many single particle measurement. This usually inefficient measurement paradigm might be a problem in frontier Transmission Electron Microscopy for example when imaging dose sensitive material or addressing very fast phenomena. However a distinctive trait of quantum mechanics is that by changing the measurement basis we can obtain a measurement where the physical quantities are optimally extracted, often by turning a phase measurement into an amplitude. Our recent demonstration of an Orbital Angular Momentum Sorter for electron (holographic or electrostatic) is a proof of this principle.
Applications:
- Single protein detection
- EMCD (electron magnetic dichroism) at atomic resolution
- Plasmonics
Image adapted from Phys. Rev. Lett. (2021)
People: Prof. S. Frabboni, Prof. Marco Beleggia, Dr. G. Gazzadi (CNR), Dr. V. Grillo (CNR), Dr. E. Rotunno (CNR), Dr. G. Bertoni (CNR)
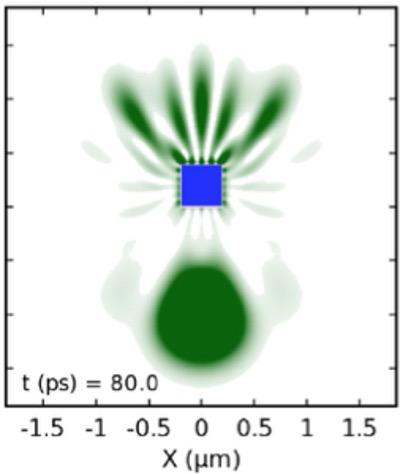
Designing devices to perform logic operations for quantum information/communication requires the ability to model and simulate the quantum evolutions of the qubits including the complexities of the realistic devices which may introduce several unwanted effects.
The group has a strong tradition in theoretical and numerical modelling of quantum states and transport of correlated charges in semiconductor nanodevices. Such studies involve both theoretical developments and High-Performance-Computing techniques. Two examples are shown in the figures. In the top panel two electron wavepackets are travelling in an electron interferometer from sources S1, S2 to detectors D1, D2, interacting in the central region. In the bottom panel, an exciton –a Coulomb interacting electron-hole pair- wavepacket (green) bounces off an anti-dot, a repulsive potential region for the electron (blue) in a so-called excitronic device. The complex correlated motion of the two particles produces a scattered wavepacket resembling a diffraction pattern (where no slit is present, though).
Realistic devices are negatively influenced by phase disruption, due to a variety of mechanisms. In this respect, quantum walks methods are used by the group to understand their influence on the unitary evolution in logic gates.
Long lived spin excitations may encode quantum information and may serve e.g. in Majorana-fermions-based quantum hardware. Such excitations strongly depend on (and can be manipulated by) device engineering. Such aspects are theoretically investigated by k.p theories in collaborations with partner experimental groups.
Images adapted from Phys. Rev. B 99, 245415 (2019) and Superlattices and Microstructures 108, 73 (2017).
People: Prof. G. Goldoni; Dr. P. Bordone, Dr. M. Govoni; Dr. A. Bertoni (CNR).
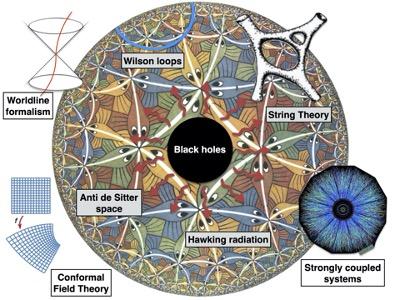
Quantum field theory (QFT) is a powerful language that allows for the description of countless phenomena, from the fundamental interactions that comprise the Standard Model of particle physics to the complex systems studied in condensed matter theory. This framework can be enlarged to also include gravity, by assuming that the elementary constituents of Nature are not point-like particles, but tiny vibrating strings. This is the main postulate of string theory, currently our best candidate for a unifying theory of all physical laws.
The group at UniMORE is interested in different aspects of QFT and string theory. One line of research focuses on the world-line description of QFT, which provides a convenient representation of large classes of QFT scattering processes in terms of simple relativistic particle models. A second line of research is the interface between gauge theories and gravity, the so-called gauge/gravity duality, or holography, which has been responsible for enormous progress in our understanding of quantum gravity, string theory itself and other areas of physics such as quantum matter and strongly coupled systems.
Image by Diego Trancanelli on top of “Circle Limit III” by M.C. Escher
People: Prof. Olindo Corradini, Prof. Diego Trancanelli, Dr. Enrico Bertuzzo
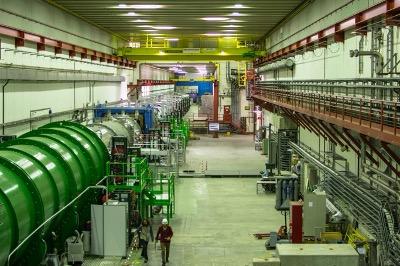
Our present knowledge of elementary particles and their interactions is well represented by a theory, the Standard Model of particle physics. However, there is a strong evidence that the Universe contains a kind of gravitationally-interacting matter (the so called "dark matter"), not composed by Standard Model particles. Experiments at large particle accelerators look for new non-Standard-Model particles and probe possible deviations from Standard Model predictions.
Research at FIM is carried out within the international collaborations NA62 and LHCb operating two large experiments at CERN accelerators and in close contact with research groups of Istituto Nazionale di Fisica Nucleare (INFN).
Data collected by these experiments are used to investigate many interesting processes in particle physics. In particular, NA62 studies very rare decays of the K+ meson, while LHCb explores particles containing heavy quarks, matter-antimatter asymmetries and multi-quark hadrons (tetra- and penta-quarks) are studied by LHCb.
Our group is especially interested in the search for new particles, antimatter production in high-energy proton-nucleus collisions and in developing detectors for future upgrades of the experimental apparata.
Image courtesy of CERN
Research Staff: Prof. A. Bizzeti